Therapeutic strategies for peripheral nerve injuries: FK506 and electrostimulation
Abstract
Efficacious therapeutics for peripheral nerve injuries remain incompletely described in the literature. However, over the last several decades, delivery of FK506 (Tacrolimus) and electrostimulation have demonstrated great promise for supplementing surgical advances in treating peripheral nerve injuries. This review describes the discovery, mechanistic investigations, and clinical translation of these strategies to promote functional recovery. FK506 has demonstrated the ability to increase the regeneration rate after nerve injury by a variety of hypothesized mechanisms, yet clinical utility remains limited due to systemic immunosuppression. Local administration of FK506 continues to be an active area of inquiry for minimizing side effects while maintaining its neuroregenerative effects. Electrostimulation of a nerve proximal to the site of surgical nerve repair has demonstrated increased axonal regeneration and accelerated recovery of both motor and sensory nerves. In addition, electrostimulation also appears to improve axon matching during reinnervation from motor to motor and sensory to sensory pathways and is used clinically in our surgeries. However, the specific parameters to best incorporate electrostimulation into the operating theater are still evolving. Utilizing translational rodent and murine models, surgical techniques and these therapeutic strategies have gradually become more viable as safety profiles and mechanisms are gradually understood. This review presents the state of the field for these therapeutic avenues and discusses further areas of research.
Keywords
INTRODUCTION
The treatment of peripheral nerve injuries has grown significantly over the last several decades as scientific discovery and mechanistic understanding of peripheral nerve regeneration have led to surgical advances. Causes of peripheral nerve injuries are wide-ranging, with multiple potential causes ranging from sharp traumatic injuries to gradual systemic degeneration. Of the over 400,000 traumatic injuries to the extremities each year in the United States, 2.3% of these patients are diagnosed with a peripheral nerve injury[1,2]. Furthermore, a significant fraction (10%-15%) of diagnosed peripheral nerve injuries are iatrogenic in nature[3].
Compared to their counterparts in the central nervous system, peripheral nerves have the capacity to regenerate and restore function to their distal end targets[4]. These cumulative injuries lead to an estimated 50,000 nerve repairs performed each year in the United States alone[5,6]. However, complete functional recovery following peripheral nerve injury is uncommon even after nerve surgery techniques such as nerve grafting and nerve transfers are performed[7-9]. After severe peripheral nerve injuries, Wallerian degeneration ensues where nerve fibers distal to the injury undergo degradation, and phagocytosis of these now-disconnected axons from the cell body by infiltrating macrophages and Schwann cells occurs[10,11]. To fill in this nerve gap, peripheral nerves regenerate at an average rate of 1mm per day and may have to traverse relatively long distances to reinnervate their target muscle fibers or sensory organs. Importantly, this rate applies to the earliest “pioneer” axons. Depending on the location of the nerve injury, recovery, even after a surgically optimized repair, can be delayed through the downregulation of the neuroregenerative factors secreted by Schwann cells[12,13]. Furthermore, denervated target organs may undergo irreversible fibrosis and atrophy over time and become unresponsive by the time the regenerating axonal front reaches the target[14,15]. In particular, both motor end plates and sensory receptors only remain viable for 12-18 months after denervation[16]. The pathway across nerve grafts itself is also affected by the length and time of denervation, as Schwann cells become senescent and no longer support reinnervation[14,17-19]. To emphasize the importance of timely nerve reconstruction and the need for neurotherapeutic strategies, delayed regeneration can result in diminished muscle mass and function[17,19].
New therapeutics and surgical techniques are needed to improve repair and recovery in these patients. Translational studies using animal models have been a major aid to innovations that have now made their way to the operating room and clinical arena. In particular, this review will survey the known landscape of FK506 (Tacrolimus) and electrostimulation as two strategies that hold great promise in aiding nerve regeneration [Figure 1A-C].
Figure 1. Increasing the rate of axonal regeneration (FK506) and the number of first-arriving pioneering axons (electrostimulation) improves function through different mechanisms. (A) A regenerating nerve illustrated by a bicycle race with each axon represented by a single cyclist and the finish line representing a suture line of previous repair. While there are some front-runners, representing pioneer axons, each cyclist travels at approximately the same speed (20 mph); (B) The addition of FK506 to the nerve regenerative environment has been shown to increase the speed of axonal regeneration. This would be as if the speed of the group of cyclists increased globally (to 30 mph), but the position between front-runners (pioneer axons) and followers is maintained; (C) The use of electrostimulation in a regenerating nerve has been proposed to promote a large number of axons to grow across a repair site more quickly rather than pioneer axons crossing the repair site with trailing axons to follow. Here, this phenomenon is represented by the pack of cyclists crossing the finish line (suture site) in a more coordinated manner, while the overall speed is maintained relative to no intervention.
FK506 (TACROLIMUS) FOR PERIPHERAL NERVE INJURY
Early studies
FK506 (Tacrolimus) is an immunosuppressive macrocyclic lactone isolated in 1984 and is now approved by the FDA for use in preventing immune rejection after organ transplantation[20,21]. Compared to the cyclosporine that was previously used for immunosuppression in solid organ transplantation, FK506 has been preferentially used given its greater efficacy and comparatively improved side effect profile, and reduced nephrotoxicity[22]. Its immunosuppressive functions are mediated by downregulating T cell-associated transcription factors like NFAT, resulting in transcriptional inhibition of T cell activation[23]. Interestingly, just several years after FK506 was discovered, several research groups elucidated its pro-neuroregenerative effects in vitro and in vivo. Using cultured neurons and sensory ganglia, treatment with FK506 stimulated neurite outgrowth[24]. Moving on to the rodent model, FK506 treatment after a rat sciatic nerve crush injury stimulated nerve regeneration with faster recovery of motor and sensory function compared to saline-treated rats[25]. Gold credits his discovery of the effect of FK506 to enhance nerve regeneration on his serendipitous observation and experience of surprising neurological recovery in a family member being treated with FK506 for an unrelated issue.
Mechanism of action
The mechanism of FK506-mediated neurotrophic effects appears to be separate from its immunosuppressive effects. Immunosuppression is believed to be mediated by FKBP-12 (FK506-binding protein 12), which, when bound by FK506, is a potent calcineurin inhibitor that ultimately prevents translocation of NFAT into the nucleus. NFAT is then unable to upregulate interleukin-2 expression, and the cellular immune cascade is suppressed[26,27]. Some studies have implicated a role for the FK506-FKBP-12 axis in nerve regeneration, with evidence that calcineurin inhibition prevents the inactivation of GAP-43[24]. GAP-43 is a protein with important functions in stimulating the nerve growth cone by interacting with actin microfilaments to promote axonal sprouting[28-30].
More recent development of non-immunosuppressive FK506 variants indicates that the major neurotrophic function of FK506 is separate from the FKBP12 mediation as FKBP12 mRNA expression was not upregulated during FK506 neuroprotection[31]. On the other hand, multiple mechanisms for the neurotrophic mechanism of FK506 are possible[32]. FKBP-52 appears to play a major role in mediating FK506 action since the FK506 neurotrophic effect is dependent on functioning FKBP-52[33]. FKBP-52 has well-described roles in cytoplasmic microtubule shuttling and closely interacts with steroid receptors, suggesting that steroid receptor signaling and intracellular transport may play important roles in peripheral nerve regeneration[34].
FK506 side effects
The clinical applications of FK506 remain limited since its mode of delivery has traditionally been systemic administration with an ensuing severe side effect profile. Among these include nephrotoxicity, hyperglycemia, and central nerve system effects that can manifest as headache, nausea, seizures, and tremor[35,36]. Prolonged weight loss secondary to diarrhea and other gastrointestinal disturbances has also been reported[25]. Given its role as an immunosuppressant, prolonged use of tacrolimus results in greater risks of infection (opportunistic infections) and cancer (skin and lymphoproliferative malignancies) from decreased immune monitoring. This adverse side effect profile requires the optimization of drug dosing, timing, and mode of delivery for tacrolimus to be used in peripheral nerve injury.
FK506 administration
For transplant patients, tacrolimus dosing is carefully modulated to maintain a steady-state tacrolimus trough levels of 5.0-15.0 ng/mL depending on the transplant organs involved and other immunosuppressive agents given that corresponds to human doses of roughly 0.1-0.2 mg/kg/day[37]. Interestingly, translational studies have demonstrated that FK506 may have multiple dose levels to implement improved neuroregeneration. When investigating 6 different levels of tacrolimus dosing after mouse sciatic nerve crush injuries compared to saline controls, the intermediate doses had no efficacy in improving regeneration rate, implying some bimodal dose efficacy[29]. To examine if improved nerve regeneration could take place at sub-immunosuppressive doses of FK506, full-thickness skin grafts from a different rat species were performed, and efficacious doses of 0.5 and 1 mg/kg/day of FK506 improved tibial nerve regeneration after transection and repair also resulted in concomitant complete rejection of the skin graft[38]. These findings raise the clinical question as to whether these sub-immunosuppressive doses of FK506 could be administered to peripheral nerve injury patients and ameliorate the adverse side effect profile. The senior author has used this drug with a small number of patients with autograft reconstruction, treating them with FK506 for one year with no systemic complications and excellent neurological recovery (unpublished). The medication timing schedule and appropriate dosing in these cases are patient-specific, given the pharmacokinetics, pharmacodynamics, and multiple drug-drug interactions that require close coordination with our immunology and transplant medicine colleagues.
The advent of upper extremity transplantation has thus allowed an opportunity to assess the neuroregenerative potential of tacrolimus in a clinically-indicated setting. The first hand transplantation performed in America was noted for more rapid nerve regeneration assessed by Tinel’s sign than previously expected from hand replants with an average regeneration rate of 2 mm/day[39]. With nerve coaptations performed 15 cm proximal to the wrist crease, Tinel’s signs were present at the fingertips within 6 months, having traversed a > 30 cm distance. A European group went on to report that in their hand transplantation with nerve coaptations 21 cm proximal to the wrist crease, Tinel’s sign had advanced to the wrist crease within 3 months (> 2 mm/day)[40]. Building upon this work, tacrolimus was found to be beneficial in utilizing nerve allograft transplantation with nerves prepared from consenting immunologically matched donors. These nerves were transplanted into patients with long peripheral nerve gaps 12-37 cm in length that were too long to be reconstructed using traditional nerve autografts. Nerve regeneration rates in these 7 patients receiving nerve allografts with immunosuppression were almost 2 mm/day[41]. The increased nerve regeneration rate of FK506 is demonstrated in [Figure 1].
To assess whether tacrolimus had any effect on promoting nerve regeneration after peripheral nerve injury and subsequent repair, a small Phase II trial was undertaken to evaluate the safety and efficacy of tacrolimus administered for two months after nerve repair[42]. Results in this study of five patients were inconclusive because although they all tolerated tacrolimus without any adverse effects and Tinel’s sign progression was faster than expected, motor recovery was incomplete, and sensory two-point discrimination was greater than >10 mm in all patients. Therefore, no beneficial effect of FK506 on peripheral nerve regeneration following repair was reported by the authors. However, the patient cohort had a heterogeneous range of nerve injuries with different types of nerves involved and various nerve repair techniques, from primary repair to unreported lengths of nerve grafting. Furthermore, inclusion criteria ranged widely from primary nerve repair within 1 week of injury and nerve grafting up to 4 months of injury. It thus remains possible that FK506 may be helpful when administered more effectively or for the optimal dose or duration.
Timing of administration
Since immediate dosing of tacrolimus at the time of injury is not clinically practical, many studies have attempted to determine effective windows of administering FK506 after injury and any surgical repair. When FK506 treatment was delayed by 3 days or 5 days following rat tibial nerve injury and repair, all timing groups demonstrated improved neuroregeneration compared to the untreated control[43]. However, the effect size of the tacrolimus therapeutic benefit did diminish with time. To further demonstrate the temporal relationship between injury, repair, and FK506 treatment, another rat study grouped rats into four cohorts of immediate repair +/- tacrolimus and 7-day delayed repair +/- tacrolimus found that delays in both repair and tacrolimus treatment were associated with suboptimal recovery[44]. Interestingly, priming of the involved rat by administering FK506 prior to nerve injury and repair appears to initiate regenerative mechanisms that result in improved functional recovery[32]. Clinically, this would be useful since many nerve transfer surgeries are performed on an elective basis, and priming the donor nerve for regeneration through the impending nerve coaptation may facilitate improved surgical outcomes.
Method of administration
Given the systemic side effects from FK506 treatment, much attention has been brought to developing targeted delivery of tacrolimus to only the site of nerve injury[45]. First, topical application of tacrolimus to the area of facial nerve repair in rabbits found that local deposition of FK506 resulted in improved nerve regeneration of the buccal branch with spontaneous movement and the highest functional score and greatest recovery upon histomorphometric analysis[46].
Other methods with controlled sustained local release of FK506 have made use of fibrin hydrogels with embedded FK506-containing microspheres. Utilizing the rat sciatic nerve cut-and-repair model, this hydrogel delivery system released FK506 for 28 days and resulted in a significant increase in regeneration of sensory and motor axons compared to the control groups[47]. For side-effect mitigation, FK506 was only detected at the sciatic nerve repair site and spinal cord, demonstrating successful sequestration away from other organs and potentially obviating systemic immunosuppression and toxicity.
Having been popularized as a method of bridging small nerve gaps without requiring nerve autografting, nerve conduits represent a potential mode of drug delivery to enhance nerve regeneration. Various nerve conduits made of natural polymers like chitosan and synthetic polymers like polytetrafluoroethylene (PTFE) have demonstrated efficacy in controlled diffusion of FK506 when implanted across nerve gaps
ELECTROSTIMULATION FOR PERIPHERAL NERVE INJURY
Early studies
The potential role of electromagnetic fields to interact with regenerating cells was first described in the early 1900s as nerve growth in cell culture exposed to electric fields was observed[50]. Subsequent studies characterized cellular changes such as nucleolar enlargement and depletion of Nissl bodies, indicative of the cellular response to axonotmesis and preparation for regeneration, after electrostimulation to peripheral nerves[51,52]. Further electrical studies of dorsal root ganglion cells of various species found that neurons would preferentially align to face the cathode and sprout new axons with greater branching towards the cathode[53,54]. Given these studies in the normal uninjured nerve, attention was turned towards the role of electrostimulation in injured nerves.
This work was expanded in animal models, starting with Hoffman, who demonstrated increased nerve sprouting at partially denervated muscles when electrostimulation was applied for 10-60 min[51,55]. Others were able to show improved muscle twitch and contractile strength recovery after nerve injury when continuous electrostimulation was applied[55,56]. Likewise, quicker recovery of the toe flexion reflex with electrostimulation, in some cases with as little as 5 min of electrostimulation therapy, was seen by Pockett and Gavin[57]. Pulsed electromagnetic stimulation following rat median nerve transection injury was found to improve resulting axon counts and nerve conduction[58]. Improved functional recovery in rabbits following soleus nerve crush injury after continuous electrostimulation with implanted nerve electrodes resulted in muscle twitch and force contraction significantly earlier than in unstimulated nerves[56]. Electrostimulation also increased the rate of central nervous system regeneration with improved functional parameters following spinal cord hemisection in both guinea pigs and dogs[59,60]. Fluorescent dye labeling of motor neurons showed that a single treatment of 1-hour electrostimulation led to accelerated axonal regeneration of repaired femoral nerve transection by 3 weeks compared to 8-10 weeks in sham-stimulated rats[61]. Additionally, electrostimulation appeared to increase the accuracy of targeted axonal regeneration with motor neurons preferentially regenerating into motor end-targets.
Mechanism of action
Complete signaling pathways following electrostimulation remain incompletely elucidated, but it is known that electrostimulation induces the expression of regeneration-associated genes necessary for axonal regeneration, such as the previously described GAP-43, brain-derived neurotrophic factor (BDNF) encoded by the BDNF gene, and its receptor trkB[62]. BDNF is a highly conserved developmental gene strongly expressed in motor neurons that plays a central role in signal transduction via the cyclic AMP pathway[12]. By maintaining cAMP by BDNF-mediated phosphodiesterase inhibition, the Rho signaling pathway is inhibited and tubulin cytoskeleton assembly is enhanced to promote nerve regeneration[63,64].
Electrostimulation also appears to induce effects outside of the neuron by exerting influence on Schwann cells. In vitro experiments on cultured Schwann cells found that an induced electrical field caused a greater than 4-fold increase in nerve growth factor (NGF) and increased cellular proliferation that was mediated by calcium signaling[65]. Sciatic crush injuries in the rat model not only found increased axonal regeneration mediated by BDNF but also earlier and increased myelination by Schwann cells[66]. Industry efforts to produce such specific upregulation of these neuroregenerative pathways have been challenging at best, demonstrating the need for clinical translation of electrostimulation into the operating rooms and clinics[67].
Electrostimulation parameters
While most of the experimental studies studied immediate electrostimulation following immediate nerve injury and repair, clinical practice does not fit that experimental picture since any surgical repair likely occurs at some delayed time following the injury. Electrostimulation performed during delayed nerve repair three months after injury found that axonal regeneration was still significantly increased[68]. Furthermore, when functional outcomes were assessed five months after electrostimulation, motor strength as measured by twitch and contraction force was similar to the immediate repair and electrostimulation group.
The most commonly utilized duration of electrostimulation in animal studies has been one hour at 20 Hz frequency; however, intraoperative stimulation of this duration would add significant financial and time expenditures to nerve repair surgeries. To test whether a shorter duration of electrostimulation would still be efficacious, ten-minute periods with a 2 mA current and 100-us pulse width were compared to one-hour periods in a rat study. Using the tibial nerve transection model, the shorter time period was found to still improve nerve regeneration and was not inferior to the one-hour duration [Figure 3A-C][69]. When the same parameters were utilized on a propensity-matched cohort of patients undergoing cubital tunnel release, the electrostimulation group demonstrated significant improvement in the Disabilities of the Arm, Shoulder, and Hand (DASH) questionnaire by 11.7 points[70]. At our institution, electrostimulation is usually applied intra-operatively directly on the nerve surface with a blunt electrode probe[71].
Figure 3. Experimental efficacy of electrostimulation and tacrolimus (FK506) in nerve regeneration. (A) GFP+ axon outgrowth was observed 14 days after repair. Electrostimulation (ES) groups displayed GFP+ axon growth that appeared more organized and unidirectional, with long axon projections extending through the distal nerve. Yellow arrows indicate the repair site; white scale bar represents 1 mm; (B) GFP+ axon outgrowth was quantified using GFP+ axon density measured at discrete spatial regions distal to the repair site. All data represented as mean ± SD, n = 4 per group. * indicates P < 0.05 10 min ES vs. 0 min ES, # indicates P < 0.05 60 min ES vs. 0 min ES, ^ indicates P < 0.05 10 min ES vs. 60 min ES. The dotted line indicates the average GFP+ density of the proximal nerve. (Adapted from Roh et al., 2022)[69]; (C) Rate of regenerating axons after crush injury in mice treated with FK-506. Taking the difference between the length measurements at each timepoint via in vivo serial transcutaneous imaging, an average rate of axonal regeneration was calculated for each experimental group. Both FK-506 preload groups exhibited a rate of regeneration that was significantly greater than all other experimental groups. The rate of regeneration in the control group at ~3 mm/day is consistent with reported rates in the literature. (P < 0.005 for comparison between indicated experimental groups) (Adapted from Yan et al., 2011)[79].
Clinical trials
Based on these reproducible animal studies, the first randomized controlled clinical trial with 21 patients enrolled to treat severe carpal tunnel syndrome by carpal tunnel release with and without intraoperative electrostimulation was reported in 2010[72]. Using a 20 Hz 1-hour stimulation protocol, electrostimulation patients demonstrated accelerated target reinnervation with complete reinnervation of thenar muscles after 12 months compared to the control group. In 2015, a double-blinded randomized controlled clinical trial of 31 patients with digital nerve transections was reported. These patients underwent surgical repair and received 20 Hz 1-hour electrostimulation or sham n-stimulation in the post-op recovery room[73]. Notably, the electrostimulation group all reported normal values of two-point discrimination and Semmes-Weinstein monofilament (indicating sensory nerve reinnervation of sensory organs) at 6 months follow-up, while these values were still abnormal in the sham n-stimulation group. However, there was no significant difference in functional recovery as reported by the DASH patient questionnaire.
In 2018, a double-blinded randomized controlled trial was reported on 54 head and neck cancer patients scheduled to undergo a surgical Level IIb +/- Level V neck dissection where retraction of the spinal accessory nerve is commonly performed and skeletonizing the nerve for lymphatic tissue is often necessary. To treat putative traction injury to the spinal accessory nerve and prevent shoulder weakness and scapular winging, these patients received 20 Hz 1-hour electrostimulation or sham stimulation intra-operatively while other portions of the surgical procedure were ongoing[74]. This trial was the first to report functional improvement with electrostimulation as these patients reported approximately only 25% of the functional shoulder impairment described by the sham stimulation cohort as measured by the Constant-Murley Shoulder score at one year following surgery. More recently, a double-blinded randomized controlled trial on 31 patients with severe cubital tunnel syndrome was published where the ulnar nerve was treated with 20 Hz 1-hour electrostimulation or sham stimulation immediately after cubital tunnel release[75]. The electrostimulation cohort reported significantly greater functional improvement at one year and significantly more motor unit potentials as detected by nerve conduction studies at three years. Representing the two most common compression neuropathies, carpal tunnel syndrome and cubital tunnel syndrome, clinical trials demonstrate that electrostimulation has efficacy in improving outcomes in chronic neuropathy[72,75].
Future directions
Currently, there are several ongoing clinical trials assessing the efficacy of electrostimulation following nerve transfers, various electrostimulation devices, and optimizing electrostimulation parameters such as the shorter duration periods as previously discussed[55,76]. Interestingly, electrostimulation has recently been compared to tacrolimus treatment, and as expected, both the electrostimulation and tacrolimus groups demonstrated robust improvement in function and increased number of regenerated myelinated axons compared to controls[77]. These findings are intriguing given the similar nerve regeneration phenotypes but with different mechanisms of action. When electrostimulation and tacrolimus were given as combination therapy in a recent study, the combination therapy was significant for achieving early functional recovery based on the grid-walk assessment and increased muscle mass compared to any of the single treatments[78]. Additionally, while the effect size of the combination treatment was roughly similar to the single treatments, the variance of the combination results in behavioral analyses was smaller than any of the treatment groups. Implications of this study suggest that these combined therapeutics may provide more reliable and consistent outcomes, and clearly, further investigations are required to optimize therapies for nerve regeneration. [Figure 2] indicates further areas of interest.
CONCLUSION
Functional recovery after nerve injury is often unsatisfactory, even after timely and appropriate surgical intervention. FK506 and electrostimulation remain two promising therapeutics to augment outcomes and improve recovery. Despite different proposed mechanisms of action, each therapeutic has been shown to improve neuroregeneration in data driven largely by small animal models and in vitro experiments [Table 1]. These models have laid the groundwork for translation to the clinical realm, mainly in assisting in the standardization of protocols for each therapeutic. For electrostimulation, it has been shown first that 1 h of stimulation produces results equal to 24 h, and subsequently that 20 min produces non-inferior results to 1 h For FK506, it has been proposed that its mechanism of neuroregeneration is distinct from that of immunosuppression, and thus a sub-immunosuppressive dose may suffice to augment regeneration without unnecessary side effects. Each of these findings brings the field one step closer to optimal translation to the clinical setting. However, there is still much to be discovered about these potential clinical therapeutic options. Further elucidation of clinical benefits in humans must be shown with more thorough clinical trials. While electrostimulation has already proven to have benefits in some clinical trials, the use of FK506 has not yet been backed up by clinical data. Appropriate patient selection will be key in studying FK506 in humans going forward to assess for clinical benefit. Ongoing and future clinical trials will build on this body of work to improve surgeons’ use of these adjunct treatments and reveal how nerve regeneration can best be augmented.
Summary of mechanism and experimental outcomes with tacrolimus (FK506) and electrostimulation
Tacrolimus (FK506) | Electrostimulation | Combined | |
Mechanism | Upregulated heat shock proteins | Increased calcium influx | |
Upregulated GAP-43 | Upregulated GAP-43 | ||
Upregulated MAPK | Upregulated microtubule assembly | ||
Increased axonal growth | Increased axonal crossing of coaptation | ||
Experimental
outcomes | Most improved histologic regeneration | Improved histology | Improved histology |
Improved walking-track recovery | Improved walking-track recovery | Earlier improved walking-track recovery, decreased variance | |
No change from control | Improved grid-walk assessment | Improved grid-walk assessment | |
Increased muscle mass | No change from control | Increased muscle mass |
DECLARATIONS
Authors’ contributionsMade substantial contributions to conception, literature review, study interpretation, and manuscript writing: Chi D, Silverman EC, Marsh EB, Wood MD, Mackinnon SE
Availability of data and materialsNot applicable.
Financial support and sponsorshipNone.
Conflicts of interestAll authors declared that there are no conflicts of interest.
Ethical approval and consent to participateNot applicable.
Consent for publicationNot applicable.
Copyright© The Author(s) 2023.
REFERENCES
1. Padovano WM, Dengler J, Patterson MM, et al. Incidence of nerve injury after extremity trauma in the United States. Hand 2022;17:615-23.
2. Taylor CA, Braza D, Rice JB, Dillingham T. The incidence of peripheral nerve injury in extremity trauma. Am J Phys Med Rehabil 2008;87:381-5.
3. Kretschmer T, Antoniadis G, Braun V, Rath SA, Richter HP. Evaluation of iatrogenic lesions in 722 surgically treated cases of peripheral nerve trauma. J Neurosurg 2001;94:905-12.
4. Fu SY, Gordon T. The cellular and molecular basis of peripheral nerve regeneration. Mol Neurobiol 1997;14:67-116.
6. Evans GR. Peripheral nerve injury: a review and approach to tissue engineered constructs. Anat Rec 2001;263:396-404.
7. Novak CB, Anastakis DJ, Beaton DE, Katz J. Patient-reported outcome after peripheral nerve injury. J Hand Surg Am 2009;34:281-7.
8. Ciaramitaro P, Mondelli M, Logullo F, et al. Italian Network for Traumatic Neuropathies. Traumatic peripheral nerve injuries: epidemiological findings, neuropathic pain and quality of life in 158 patients. J Peripher Nerv Syst 2010;15:120-7.
9. Ray WZ, Mackinnon SE. Management of nerve gaps: autografts, allografts, nerve transfers, and end-to-side neurorrhaphy. Exp Neurol 2010;223:77-85.
10. Gaudet AD, Popovich PG, Ramer MS. Wallerian degeneration: gaining perspective on inflammatory events after peripheral nerve injury. J Neuroinflammation 2011;8:110.
11. Jessen KR, Mirsky R. The repair Schwann cell and its function in regenerating nerves. J Physiol 2016;594:3521-31.
12. McGregor CE, English AW. The Role of BDNF in peripheral nerve regeneration: activity-dependent treatments and Val66Met. Front Cell Neurosci 2018;12:522.
13. Eggers R, Tannemaat MR, Ehlert EM, Verhaagen J. A spatio-temporal analysis of motoneuron survival, axonal regeneration and neurotrophic factor expression after lumbar ventral root avulsion and implantation. Exp Neurol 2010;223:207-20.
14. Gordon T, Tyreman N, Raji MA. The basis for diminished functional recovery after delayed peripheral nerve repair. J Neurosci 2011;31:5325-34.
15. Moore AM, Wagner IJ, Fox IK. Principles of nerve repair in complex wounds of the upper extremity. Semin Plast Surg 2015;29:40-7.
17. Kobayashi J, Mackinnon SE, Watanabe O, et al. The effect of duration of muscle denervation on functional recovery in the rat model. Muscle Nerve 1997;20:858-66.
18. Pellegrino RG, Spencer PS. Schwann cell mitosis in response to regenerating peripheral axons in vivo. Brain Res 1985;341:16-25.
19. Weinberg HJ, Spencer PS. The fate of Schwann cells isolated from axonal contact. J Neurocytol 1978;7:555-69.
20. Kino T, Hatanaka H, Hashimoto M, et al. FK-506, a novel immunosuppressant isolated from a Streptomyces. I. Fermentation, isolation, and physico-chemical and biological characteristics. J Antibiot 1987;40:1249-55.
21. Starzl TE, Todo S, Fung J, Demetris AJ, Venkataramman R, Jain A. FK 506 for liver, kidney, and pancreas transplantation. Lancet 1989;2:1000-4.
22. The U.S. Multicenter FK506 Liver Study Group. A comparison of tacrolimus (FK 506) and cyclosporine for immunosuppression in liver transplantation. N Engl J Med 1994;331:1110-5.
23. Mouzaki A, Dai Y, Weil R, Rungger D. Cyclosporin A and FK506 prevent the derepression of the IL-2 gene in mitogen-induced primary T lymphocytes. Cytokine 1992;4:151-60.
24. Lyons WE, George EB, Dawson TM, Steiner JP, Snyder SH. Immunosuppressant FK506 promotes neurite outgrowth in cultures of PC12 cells and sensory ganglia. Proc Natl Acad Sci U S A 1994;91:3191-5.
25. Gold BG, Katoh K, Storm-Dickerson T. The immunosuppressant FK506 increases the rate of axonal regeneration in rat sciatic nerve. J Neurosci 1995;15:7509-16.
26. Grand AG, Myckatyn TM, Mackinnon SE, Hunter DA. Axonal regeneration after cold preservation of nerve allografts and immunosuppression with tacrolimus in mice. J Neurosurg 2002;96:924-32.
27. Liu J, Albers MW, Wandless TJ, et al. Inhibition of T cell signaling by immunophilin-ligand complexes correlates with loss of calcineurin phosphatase activity. Biochemistry 1992;31:3896-901.
28. Gold BG, Yew JY, Zeleny-Pooley M. The immunosuppressant FK506 increases GAP-43 mRNA levels in axotomized sensory neurons. Neurosci Lett 1998;241:25-8.
29. Udina E, Ceballos D, Verdú E, Gold BG, Navarro X. Bimodal dose-dependence of FK506 on the rate of axonal regeneration in mouse peripheral nerve. Muscle Nerve 2002;26:348-55.
30. Skene JH, Jacobson RD, Snipes GJ, McGuire CB, Norden JJ, Freeman JA. A protein induced during nerve growth (GAP-43) is a major component of growth-cone membranes. Science 1986;233:783-6.
31. Tanaka K, Fujita N, Higashi Y, Ogawa N. Neuroprotective and antioxidant properties of FKBP-binding immunophilin ligands are independent on the FKBP12 pathway in human cells. Neurosci Lett 2002;330:147-50.
32. Snyder AK, Fox IK, Nichols CM, et al. Neuroregenerative effects of preinjury FK-506 administration. Plast Reconstr Surg 2006;118:360-7.
33. Gold BG, Densmore V, Shou W, Matzuk MM, Gordon HS. Immunophilin FK506-binding protein 52 (not FK506-binding protein 12) mediates the neurotrophic action of FK506. J Pharmacol Exp Ther 1999;289:1202-10.
34. Czar MJ, Owens-Grillo JK, Yem AW, et al. The hsp56 immunophilin component of untransformed steroid receptor complexes is localized both to microtubules in the cytoplasm and to the same nonrandom regions within the nucleus as the steroid receptor. Mol Endocrinol 1994;8:1731-41.
35. Dumont FJ. FK506, an immunosuppressant targeting calcineurin function. Curr Med Chem 2000;7:731-48.
36. Felldin M, Bäckman L, Brattström C, et al. Rescue therapy with tacrolimus (FK 506) in renal transplant recipients--a Scandinavian multicenter analysis. Transpl Int 1997;10:13-8.
37. Vanrenterghem Y, van Hooff JP, Squifflet JP, et al. European Tacrolimus/MMF Renal Transplantation Study Group. Minimization of immunosuppressive therapy after renal transplantation: results of a randomized controlled trial. Am J Transplant 2005;5:87-95.
38. Yang RK, Lowe JB 3rd, Sobol JB, Sen SK, Hunter DA, Mackinnon SE. Dose-dependent effects of FK506 on neuroregeneration in a rat model. Plast Reconstr Surg 2003;112:1832-40.
39. Jones JW, Gruber SA, Barker JH, Breidenbach WC. Successful hand transplantation. One-year follow-up. Louisville hand transplant team. N Engl J Med 2000;343:468-73.
40. Owen ER, Dubernard JM, Lanzetta M, et al. Peripheral nerve regeneration in human hand transplantation. Transplant Proc 2001;33:1720-1.
41. Mackinnon SE, Doolabh VB, Novak CB, Trulock EP. Clinical outcome following nerve allograft transplantation. Plast Reconstr Surg 2001;107:1419-29.
42. Phan DQ, Schuind F. Tolerance and effects of FK506 (tacrolimus) on nerve regeneration: a pilot study. J Hand Surg Eur Vol 2012;37:537-43.
43. Sobol JB, Lowe III JB, Yang RK, Sen SK, Hunter DA, Mackinnon SE. Effects of delaying FK506 administration on neuroregeneration in a rodent model. J Reconstr Microsurg 2003;19:113-8.
44. Brenner MJ, Fox IK, Kawamura DH, et al. Delayed nerve repair is associated with diminished neuroenhancement by FK506. Laryngoscope 2009;114:570-6.
45. Daeschler SC, Feinberg K, Harhaus L, Kneser U, Gordon T, Borschel GH. Advancing Nerve Regeneration: Translational Perspectives of Tacrolimus (FK506). Int J Mol Sci 2023;24:12771.
46. Diaz LM, Steele MH, Guerra AB, et al. The role of topically administered FK506 (tacrolimus) at the time of facial nerve repair using entubulation neurorrhaphy in a rabbit model. Ann Plast Surg 2004;52:407-13.
47. Tajdaran K, Chan K, Shoichet MS, Gordon T, Borschel GH. Local delivery of FK506 to injured peripheral nerve enhances axon regeneration after surgical nerve repair in rats. Acta Biomater 2019;96:211-21.
48. Labroo P, Hilgart D, Davis B, et al. Drug-delivering nerve conduit improves regeneration in a critical-sized gap. Biotechnol Bioeng 2019;116:143-54.
49. Zhao J, Zheng X, Fu C, Qu W, Wei G, Zhang W. FK506-loaded chitosan conduit promotes the regeneration of injured sciatic nerves in the rat through the upregulation of brain-derived neurotrophic factor and TrkB. J Neurol Sci 2014;344:20-6.
50. Ingvar S. Reaction of cells to the galvanic current in tissue cultures. Experimental Biology and Medicine 1920;17:198-9.
51. Hoffman H. Acceleration and retardation of the process of axon-sprouting in partially devervated muscles. Aust J Exp Biol Med Sci 1952;30:541-66.
52. Hyden H. Protein and nucleotide metabolism in the nerve cell under different functional conditions. Symp Soc Exp Biol 1947;1:152-62.
53. Hinkle L, McCaig CD, Robinson KR. The direction of growth of differentiating neurones and myoblasts from frog embryos in an applied electric field. J Physiol 1981;314:121-35.
54. McCaig CD. Nerve branching is induced and oriented by a small applied electric field. J Cell Sci 1990;95:605-15.
55. Zuo KJ, Gordon T, Chan KM, Borschel GH. Electrical stimulation to enhance peripheral nerve regeneration: update in molecular investigations and clinical translation. Exp Neurol 2020;332:113397.
56. Nix WA, Hopf HC. Electrical stimulation of regenerating nerve and its effect on motor recovery. Brain Res 1983;272:21-5.
57. Pockett S, Gavin RM. Acceleration of peripheral nerve regeneration after crush injury in rat. Neurosci Lett 1985;59:221-4.
58. Wilson DH, Jagadeesh P. Experimental regeneration in peripheral nerves and the spinal cord in laboratory animals exposed to a pulsed electromagnetic field. Paraplegia 1976;14:12-20.
59. Borgens RB, Blight AR, Murphy DJ, Stewart L. Transected dorsal column axons within the guinea pig spinal cord regenerate in the presence of an applied electric field. J Comp Neurol 1986;250:168-80.
60. Borgens RB, Toombs JP, Breur G, et al. An imposed oscillating electrical field improves the recovery of function in neurologically complete paraplegic dogs. J Neurotrauma 2009;16:639-57.
61. Al-Majed AA, Neumann CM, Brushart TM, Gordon T. Brief electrical stimulation promotes the speed and accuracy of motor axonal regeneration. J Neurosci 2000;20:2602-8.
62. Al-Majed AA, Tam SL, Gordon T. Electrical stimulation accelerates and enhances expression of regeneration-associated genes in regenerating rat femoral motoneurons. Cell Mol Neurobiol 2004;24:379-402.
63. Han PJ, Shukla S, Subramanian PS, Hoffman PN. Cyclic AMP elevates tubulin expression without increasing intrinsic axon growth capacity. Exp Neurol 2004;189:293-302.
64. Sit ST, Manser E. Rho GTPases and their role in organizing the actin cytoskeleton. J Cell Sci 2011;124:679-83.
65. Huang J, Ye Z, Hu X, Lu L, Luo Z. Electrical stimulation induces calcium-dependent release of NGF from cultured Schwann cells. Glia 2010;58:622-31.
66. Wan L, Xia R, Ding W. Short-term low-frequency electrical stimulation enhanced remyelination of injured peripheral nerves by inducing the promyelination effect of brain-derived neurotrophic factor on Schwann cell polarization. J Neurosci Res 2010;88:2578-87.
67. de Albornoz P, Delgado PJ, Forriol F, Maffulli N. Non-surgical therapies for peripheral nerve injury. Br Med Bull 2011;100:73-100.
68. Elzinga K, Tyreman N, Ladak A, Savaryn B, Olson J, Gordon T. Brief electrical stimulation improves nerve regeneration after delayed repair in Sprague Dawley rats. Exp Neurol 2015;269:142-53.
69. Roh J, Schellhardt L, Keane GC, et al. Short-duration, pulsatile, electrical stimulation therapy accelerates axon regeneration and recovery following tibial nerve injury and repair in rats. Plast Reconstr Surg 2022;149:681e-90e.
70. Evans A, Padovano WM, Patterson JMM, et al. Beyond the cubital tunnel: use of adjunctive procedures in the management of cubital tunnel syndrome. Hand 2023;18:203-13.
71. Chi D, Ha AY, Alotaibi F, et al. A surgical framework for the management of incomplete axillary nerve injuries. J Reconstr Microsurg 2023;39:616-26.
72. Gordon T, Amirjani N, Edwards DC, Chan KM. Brief post-surgical electrical stimulation accelerates axon regeneration and muscle reinnervation without affecting the functional measures in carpal tunnel syndrome patients. Exp Neurol 2010;223:192-202.
73. Wong JN, Olson JL, Morhart MJ, Chan KM. Electrical stimulation enhances sensory recovery: a randomized controlled trial. Ann Neurol 2015;77:996-1006.
74. Barber B, Seikaly H, Ming Chan K, et al. Intraoperative brief electrical stimulation of the spinal accessory nerve (BEST SPIN) for prevention of shoulder dysfunction after oncologic neck dissection: a double-blinded, randomized controlled trial. J Otolaryngol Head Neck Surg 2018;47:7.
75. Power HA, Morhart MJ, Olson JL, Chan KM. Postsurgical electrical stimulation enhances recovery following surgery for severe cubital tunnel syndrome: a double-blind randomized controlled trial. Neurosurgery 2020;86:769-77.
76. Juckett L, Saffari TM, Ormseth B, Senger JL, Moore AM. The effect of electrical stimulation on nerve regeneration following peripheral nerve injury. Biomolecules 2022;12:1856.
77. Jo S, Pan D, Halevi AE, et al. Comparing electrical stimulation and tacrolimus (FK506) to enhance treating nerve injuries. Muscle Nerve 2019;60:629-36.
78. Marsh EB, Schellhardt L, Hunter DA, Mackinnon SE, Snyder-Warwick AK, Wood MD. Electrical stimulation or tacrolimus (FK506) alone enhances nerve regeneration and recovery after nerve surgery, while dual use reduces variance and combines strengths of each in promoting enhanced outcomes. Muscle Nerve 2023;67:78-87.
Cite This Article
Export citation file: BibTeX | RIS
OAE Style
Chi D, Silverman EC, Marsh EB, Wood MD, Mackinnon SE. Therapeutic strategies for peripheral nerve injuries: FK506 and electrostimulation. Plast Aesthet Res 2023;10:56. http://dx.doi.org/10.20517/2347-9264.2023.48
AMA Style
Chi D, Silverman EC, Marsh EB, Wood MD, Mackinnon SE. Therapeutic strategies for peripheral nerve injuries: FK506 and electrostimulation. Plastic and Aesthetic Research. 2023; 10: 56. http://dx.doi.org/10.20517/2347-9264.2023.48
Chicago/Turabian Style
Chi, David, Erin C. Silverman, Evan B. Marsh, Matthew D. Wood, Susan E. Mackinnon. 2023. "Therapeutic strategies for peripheral nerve injuries: FK506 and electrostimulation" Plastic and Aesthetic Research. 10: 56. http://dx.doi.org/10.20517/2347-9264.2023.48
ACS Style
Chi, D.; Silverman EC.; Marsh EB.; Wood MD.; Mackinnon SE. Therapeutic strategies for peripheral nerve injuries: FK506 and electrostimulation. Plast. Aesthet. Res. 2023, 10, 56. http://dx.doi.org/10.20517/2347-9264.2023.48
About This Article
Special Issue
Copyright
Data & Comments
Data
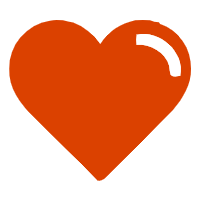

Comments
Comments must be written in English. Spam, offensive content, impersonation, and private information will not be permitted. If any comment is reported and identified as inappropriate content by OAE staff, the comment will be removed without notice. If you have any queries or need any help, please contact us at support@oaepublish.com.